Many bacteria use a flagellar motor to move in the environment. The motor consists of the stator complex containing the two components MotA and MotB with not much being known about their evolution. This aspect was explored in the study “Ancestral reconstruction of the MotA stator subunit reveals that conserved residues far from the pore are required to drive flagellar motility” in microLife. In a #BehindThePaper interview, Md Imtiazul Islam and Matt Baker explain how knowledge about the interactions of MotA and MotB helps us better understand evolution.
What excites you about studying the bacterial flagellar motor?
The bacterial flagellar motor is the motility organ that many bacteria use to move around in the environment. This self-assembling electrical rotary nanomachine can rotate up to 100,000 rpm, which is five times faster than a supercar engine. Plus, its conversion efficiency of electric-to-mechanical-torque is nearly 100%.
The bacterial flagellar motor consists of a rotor and a stator. While the rotor generates the rotation, the stator generates energy from ion transit. However, how the components of the bacterial flagellar motor evolved and adapted over time to function together as a complex, and the exact mechanisms of ion selection and rotation are not completely known.
This is why we wanted to explore the bacterial flagellar motor and its components. We specifically focused on the stator complex because it is the engine that powers the motor and drives the rotation of the flagellar filament.
What is the importance of your study for the microbiology field?
In our study “Ancestral reconstruction of the MotA stator subunit reveals that conserved residues far from the pore are required to drive flagellar motility” in microLife, we shed light on the underlying mechanisms of the bacterial flagellar motor.
In 2020, it was discovered independently by the labs of Dr Susan Lea and Dr Nicholas Taylor that the stator complex is a tiny rotating nanomachine with 5 MotA subunits rotating around a ‘stalk’ of 2 MotB subunits. With this structural information, we sought to better understand how this complex works. By characterizing and analyzing the MotA protein, we identified several regions of the stator complex that are essential for the function of the bacterial flagellar motor.
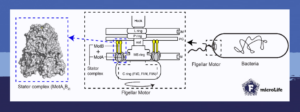
Which technique did you use for the protein analysis?
To study MotA, the stator complex of the bacterial flagellar motor, we used ancestral sequence reconstruction. This approach enabled us to learn about contemporary proteins and their structural, evolutionary and functional information. By examining the best estimates about the past, we used evolution as a guide to systematically scan through a set of proteins that will likely work.
We could then rationally test specific mutations to determine whether they disrupt or restore protein function. Hence, we showed how ancestral sequence reconstruction can inform on the structure and function of modern proteins. Based on protein sequences, we calculated a phylogenetic tree to estimate the relationship between contemporary proteins and their ancestors.
How did ancestral sequence reconstruction guide your next steps?
After determining the sequences of the ancestor proteins, we went one step further and expressed the MotA variants in E. coli to test whether the resulting flagellum would rotate. By analyzing these variants, we discerned which parts of MotA are essential and which parts do not contribute to MotA function.
We are the first to apply ancestral sequence reconstruction to the bacterial flagellar motor. In one of our previous studies, we reconstructed ancestral MotB proteins.
What are the limitations of your study?
We have only been able to reconstruct the stator complex one subunit at a time. We next hope to reconstruct both the MotA and MotB subunits of the protein together with our best phylogenetic effort.
By examining how the complex evolved together, we can work towards reconstructing and resurrecting the ancient stator complex. This will help understand how the stator can – and did – adapt and work towards its origin.
- Read the paper “Ancestral reconstruction of the MotA stator subunit reveals that conserved residues far from the pore are required to drive flagellar motility” by Islam et al. (2023) in microLife.
About the authors of this blog
Md Imtiazul Islam recently completed his PhD on ancestral bacterial flagellar motor protein reconstruction and characterization at the University of New South Wales, Sydney (Australia), under the supervision of Dr Matthew Baker. His research mainly focused on studying the structure and evolution of the stator complex MotA. Previously, he completed his Bachelor and Master of Science degrees in Microbiology in Bangladesh and a Masters by Research degree in Medical Microbiology in South Korea.
Matt Baker finished an Hons in Chemistry at the Australian National University studying Fluctuation Theorems before completing his DPhil in Physics at the University of Oxford (UK) looking at the molecular motor that makes bacteria swim. There he learned to love microbiology as he could work with complex living systems but also put them ‘on ice’ when needed. Matt started his research group in 2018 at the School of Biotechnology and Biomolecular Science at the University of New South Wales, Sydney (Australia), as a Scientia Fellow and was promoted to Scientia Associate Professor in 2023.
About this blog section
#BehindThePaper posts on the #FEMSmicroBlog aim to bring science closer to different audiences and to tell more about the scientific or personal journey to come to the results.
Do you want to be a guest contributor? |
The #FEMSmicroBlog welcomes external bloggers, writers and SciComm enthusiasts. Get in touch if you want to share your idea for a blog entry with us! |